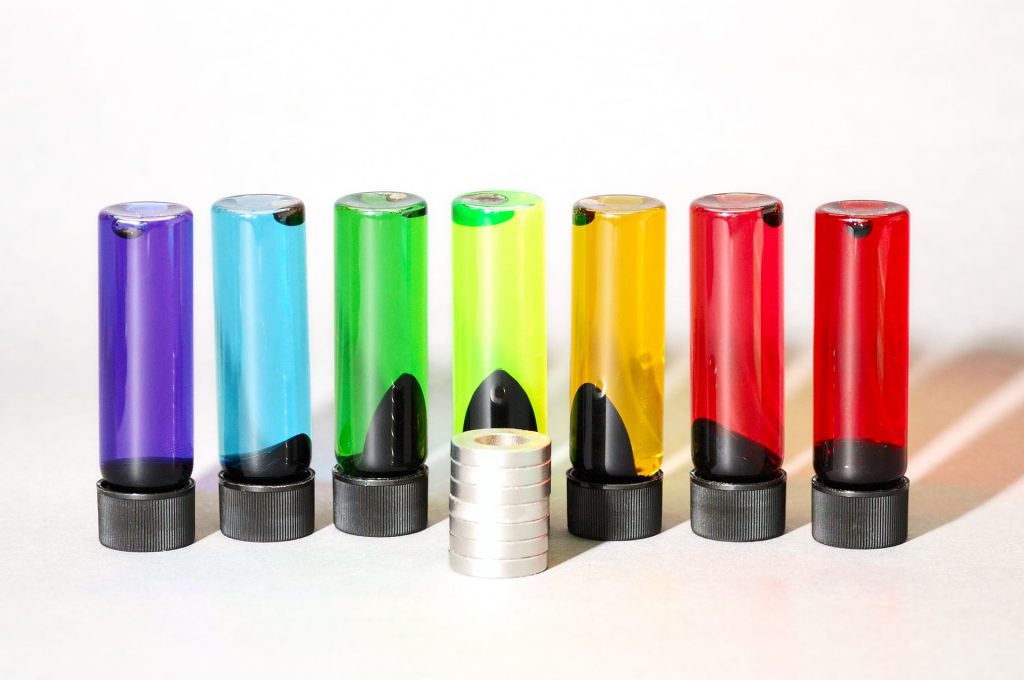
With the rapid development of nanotechnology, nanoparticles have been mass-produced and widely used in daily life. Continuous separation of nanoparticles plays a vital role in a wide range of research fields, from fundamental nanoscience to biological and environmental applications, including circulating tumor cell analysis, exosome detection, cargo loading efficiency and drug delivery, hazardous material removal, quantum dot production, and biosensor materials. However, due to the small size of nanoparticles, it is difficult to achieve high separation performance, efficiency, or even high-throughput. Therefore, the separation of nanoparticles with high purity and high resolution is still an arduous task. In the research and production of nanomaterials, it is urgent to obtain nanoparticles with certain particle size, morphology, and structure by appropriate separation methods. Here we list several commonly used technologies for nanoparticle separation.
Field flow fractionation
The field flow fractionation (FFF) was first invented by Giddings in 1966 and has become an important method for the separation of nanoparticles. FFF is a method that applies “field” to a suspension or solution in a long and narrow tunnel, acts vertically (or from other angles) in the direction of the mobile phase, and uses different mobility under the action of “field” to achieve the purpose of separation. This “field” can be the asymmetric flow field of a semi-permeable membrane, or a centrifugal field, gravity field, thermal field, electric field, and magnetic field. For example, under the action of centrifugal force, nanoparticles can be separated according to different sizes and densities. It has the advantages of a wide range of samples and high separation efficiency. This method can also be used to separate or detect nanoparticles in combination with other instruments such as dynamic light scatterers.
FFF is suitable for the detection and separation of nanoparticles in complex substrates such as atmosphere, tap water, sewage, surface water, sediment, sediment, and biological samples. According to the difference of sample diffusion coefficient, FFF can achieve continuous, high sensitivity and high accuracy separation, and complete the determination of nanoparticle size distribution at the same time. The deficiency of FFF is that the sample is easy to lose, and the pH value and ionic strength of the sample will also be changed.
Ultracentrifugation
Ultracentrifugation includes gradient ultracentrifugation based on density, viscosity, and velocity. Different methods can be selected according to the size of target nanoparticles. Density gradient ultracentrifugation is a separation method in which samples are added to inert gradient media for sedimentation, and nanoparticles of different sizes are assigned to specific positions to form different zones. This is an extensive, non-destructive, and scalable separation method. Density gradient ultracentrifugation has been successfully applied to the separation of gold nanoparticles and biological macromolecules with different chemical properties, structures, and sizes.
Ultracentrifugation can separate and enrich nanoparticles by using different properties such as weight and density and can purify chemically modified nanoparticles and separate nanoparticles with different morphologies, sizes, or aggregations. Meanwhile, it has the advantage of less sample loss and has been widely used in the separation of gold nanoparticles and quantum dots.
Membrane separation
Generally speaking, the membrane separation method is to retain the nanoparticles of different sizes through the filtration of the membrane, so as to achieve the purpose of separation. The ultrafiltration process is a pressure-driven separation method, which has the characteristics of fast separation speed and a wide range of applications and can reach the industrial scale. Compared with resin chromatography, membrane separation is more simple and efficient, easier to realize laboratory and industrial applications, and promotes the innovation of hemodialysis equipment.
The thickness, pore size, hydrophobicity, and internal surface area of the membrane are important factors affecting the separation effect and separation rate. The higher surface area will lead to the retention, loss, and blockage of the sample, and the thicker membrane will make the transport speed in the purification process too slow.
Chromatography separation
The membrane separation method is suitable for the separation of high concentration nanoparticles, while for low concentration nanoparticles, the chromatography separation method has better advantages. The characteristic of chromatography separation is that the sample can be separated and quantitatively detected at the same time. Due to the hydrodynamic and electrophoretic properties of nanoparticles, the structural changes of nanoparticles before and after surface modification can be detected by chromatography. The commonly used chromatography separation methods include high-performance liquid chromatography, size exclusion chromatography, capillary electrochromatography, and so on. The chromatographic stationary phase, mobile phase, and column can be selected according to different separation objects and purposes.
Size exclusion chromatography can separate nanoparticles according to their shape and size and has the advantages of short separation time, narrow bandwidth, high sensitivity, and low sample loss.
Magnetic separation
Magnetic separation is an effective method to separate nanoparticles by magnetic force. Magnetic separation in the form of axial flow has been developed in the early years, which can achieve the effect of efficient separation by changing the number of channels, the size of channels, the length of separators, and magnetic coils.
If nanoparticles are with similar particle sizes, it will be difficult to separate them by gradient centrifugation and ultrafiltration. Thus, the separation of specific target nanoparticles can be achieved by using selectively capturing magnetic nanoparticles. According to the surface chemical properties of the target nanoparticles, the magnetic nanoparticles with high selectivity to capture the target nanoparticles are designed and synthesized, and the two are mixed, and then the magnetic field is used to achieve the purpose of specific separation.
Hybrid-type separation
In recent years, hybrid separation technologies (dielectrophoresis-assisted, magnetophoresis-assisted, acoustophoresis-assisted and optophoresis-assisted) have been developed by combining the advantages of passive and active methods. Therefore, these hybrid methods can take advantage of the active method to ensure high throughput and additional controllability, while the passive mode ensures the ability to process multiple samples at the same time. However, the hybrid separation also has several limitations, such as additional power supply and complicated operating settings. In addition, the separation mechanism can only operate under strict conditions, such as a specific pH range or NaCl concentration range. Although the individual operating mechanism is very simple, due to the coupling of experimental parameters such as solution salinity, conductivity and viscosity, flow rate and flow pattern, pressure, etc., the combination of two or more driving forces makes the experimental operation complicated stand-up.
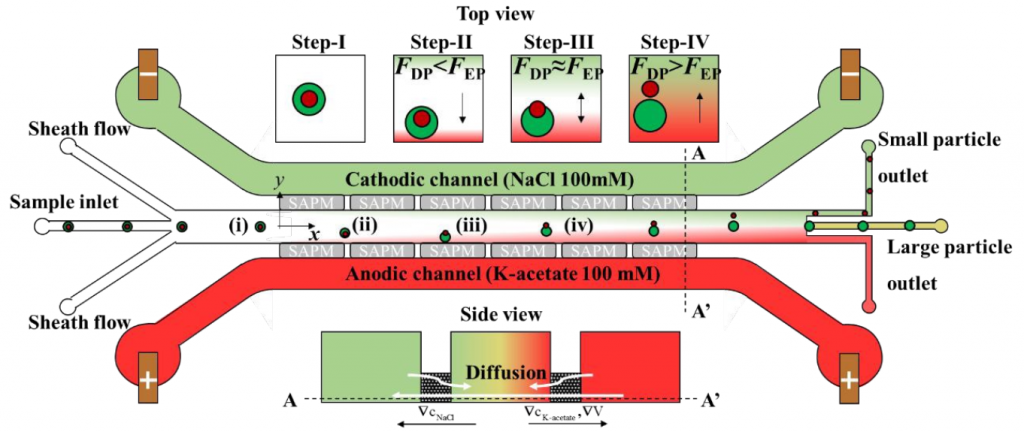
To solve this, Professor Taesung Kim (Department of Mechanical Engineering, Ulsan National Institute of Science and Technology, Republic of Korea) and his colleagues describe a new hybrid technology that combines diffusion permeation (DP) and electrophoresis (EP) to achieve the separation of nanoparticles, called low potential assisted diffusion (LEPDP) technology (Figure 1). Detailed results could be found on Lab on a Chip.
In the presence of an electrolyte concentration gradient, DP occurs spontaneously, and the diffusion mobility can be controlled by changing the type and/or strength of the buffer solution, thereby actively controlling the migration speed of the nanoparticles. In addition, EP acts on charged particles in the presence of an electric field, thereby allowing another active control of the electrophoretic mobility of nanoparticles. Therefore, due to the interaction between the concentration gradient and the electric field, LEPDP takes advantage of the advantages of DP and EP. In other words, the concentration of the electrolyte determines the conductivity of the solution, so the combination of DP and EP can play a complementary role.
They first proved that the LEPDP effect makes it possible to separate nanoparticles (such as PS, SiO2, PLA (CD Bioparticles, Shirley, NY, USA, concentration 0.05% w/v)) with different Zeta potentials. In other words, the nanoparticles with the highest zeta potential have the best separation effect because they have the fastest migration speed on the main channel, which can realize the separation of nanoparticles based on surface charge. On this basis, they proved that the same separation device can be applied to the particle size separation experiments of different PS nanoparticles with diameters of 500, 200, and 50 nm. That is to say, under the LEPDP effect, nanoparticles of different sizes show different migration speeds and change their migration directions. In addition, they conducted an off-chip analysis by extracting some separated nanoparticle samples from the chip and confirmed that the separation performance was as high as 95% for the 200 and 500 nm nanoparticles separation. Professor Kim said: “We anticipate that our multi-physics-based nanoparticle separation mechanism relying on the nonlinear but synergistic LEPDP effect would not only provide a novel nanoparticle separation technique but also shows remarkable potential for enabling sub-100-nm nanoparticle separation in the near future.”
Reference
Lee, K., Lee, J., Ha, D., Kim, M., & Kim, T. (2020). Low-electric-potential-assisted diffusiophoresis for continuous separation of nanoparticles on a chip. Lab on a Chip, 20(15), 2735-2747.